In the last episode, the electrical dialogue between cells and digital systems changed its form, taking us to a different level in the multi-layered number of scales and lenses we can choose for looking at biological systems. From the detailed dynamics of the single cell, we passed onto the consideration of a cellular network living in a two-dimensional environment. If such cells are neurons, that implies including a fundamental aspect of their nature: the exchange and computation of information through electrophysiological signals between cells.

But if we want to approach even more closely the actual nature of biological neural networks, we are definitely still missing something. In fact, in our (and other) brains, cells do not grow on a planar surface, but rather occupy a three-dimensional volume together with other cellular types with supportive or other roles. Let’s skip for now the coexistence of other cell types. If we have in mind to replicate the physiology of the CNS, growing cells in a three-dimensional environment is crucial. In fact, that allows for the formation of way more connections between neurons since they can grow their axons and dendrites in all directions. That allows for comprising more of the complexity of the natural system at a structural, and then functional level.

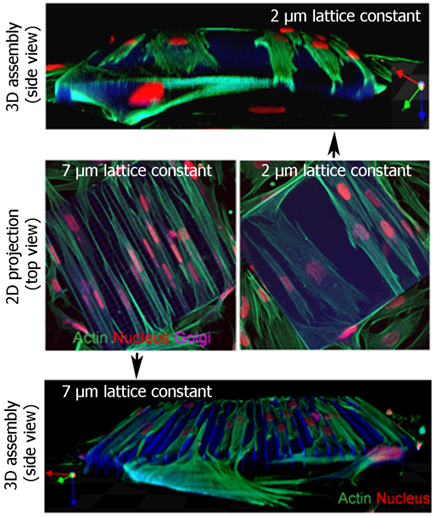
A three-dimensional support for cell culture must not only provide for a three-dimensional architectural infrastructure, but also allowing the suitable growing and living condition for the cells. That is, its surface must be biocompatible. This can be obtained with the use of specific nanostructured materials, and their biochemical functionalization.
An interesting example is the use of graphene or similar carbon-derived nanostructures for building up three-dimensional scaffolds for culturing 3D networks of biological neurons. Not only carbon-based nanostructures can be enriched with the suitable functional groups for cell growth, guidance and adhesion, but they also make for highly sensitive electrodes, since they are conductive.

That means we added a third dimension to the environment where we can grow biological neural networks in vitro. And while that has a variety of applications in the broad and diverse field of tissue engineering, considering such interface we still have a foot into the domain of electrophysiology. But this time, signals can be administered to and collected from the cells in 4D: a three-dimensional bio-hybrid system evolving and living (or not?) in time.
Roberta Bardini